Celltex News
From Totipotent to Unipotent: Understanding Stem Cell Types and Differentiation Potential
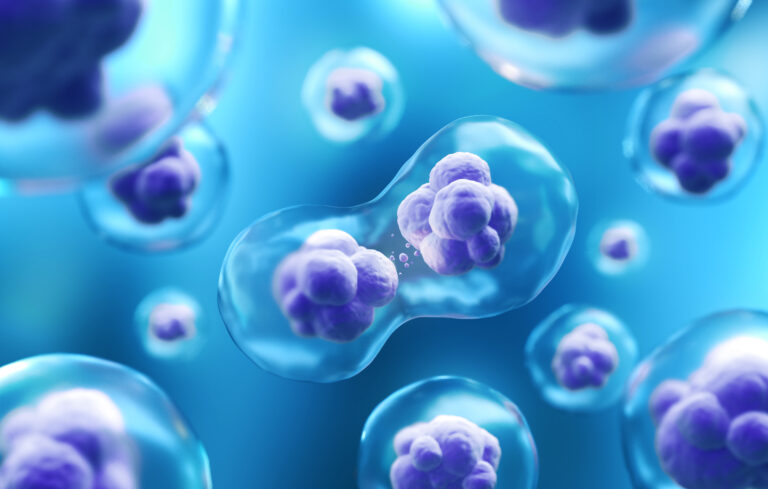
Thanks to advancements in stem cell research, the future of medicine is already here. Physicians will soon have a natural tool capable of repairing damaged tissue, regulating the immune system, and so much more.
Although stem cell therapy’s future applications and potential are still being investigated, stem cells have revolutionized the landscape of medicine, offering a promising therapy option for many conditions and diseases. As of 2022, more than 3,000 adult stem cell therapy clinical trials were registered in the World Health Organization’s International Clinical Trials Registry. [1]
However, if you’re interested in receiving stem cell therapy in the future, be aware that not all stem cells are the same. It’s important to understand the differences between various types of stem cells and which ones are utilized in regenerative medicine.
This article will explore:
- What are stem cells?
- What are the characteristics that stem cells share?
- What is stem cell potency?
- What are the different types of stem cells?
- What type of stem cell is most often used in regenerative medicine and why?
- How can you bank stem cells for future therapy?
A Brief Introduction to Stem Cells and Differentiation
Stem cells are like the body’s repair kit. They are immature cells that have yet to develop into a particular type of cell, such as cartilage, bone, nerve, or muscle cells. Stem cells possess the potential to become other cell types to repair damaged tissues. This action is called “differentiation.”
All major types of stem cells share two characteristics. Firstly, stem cells are capable of self-renewal, meaning they can proliferate in near perpetuity, generating countless identical copies of themselves. The second characteristic of stem cells is potency, which we describe, in detail, below.
Stem Cell Potency Explained
Unlike strength or power in the traditional sense, in stem cells, potency refers to the ability to differentiate into specialized cell types (cartilage, bone, skin, etc.). This versatility sets stem cells apart from specialized cells.
The level of potency determines the range of cell types that a stem cell can potentially become. Stem cells with a greater potency may differentiate into a wider variety of cell types. Stem cells with a lower potency are more limited in their differentiation potential and thus can only regenerate into specific cell types.
Stem Cell Potencies
Let’s examine each level of potency, ranging from greatest to least, before exploring the most common types of stem cells in the next section.
TOTIPOTENT
The prefix toti- comes from the Latin word totus, which means whole or entire. In the context of stem cells, totipotent refers to the cell’s ability to differentiate into all the cell types necessary to form a complete organism, including a human embryo and organs outside the embryo, such as the placenta. [2]
PLURIPOTENT
Pluripotent refers to the ability of a stem cell to differentiate into almost any cell type in the body (excluding cells of the placenta and supporting structures). Thus, pluripotent stem cells do not inherently possess as much differentiation potential as totipotent stem cells. The totipotency and pluripotency of stem cells are short-lived, occurring only during the early formation of the embryo. Nonetheless, they “establish the foundation for the development of all mammals,” explains a 2024 research review in the journal Cell Stem Cell. [3]
Totipotent and pluripotent stem cells have the potential to differentiate into a wider range of cell types. However, they also carry a higher risk of forming teratomas (a type of tumor) or other unwanted tissues in applications. Additionally, the use of totipotent and pluripotent stem cells raises obvious ethical concerns due to their potential to develop into a complete organism. [4]
MULTIPOTENT
Lower down the totem pole of stem cell potency, multipotent stem cells differentiate into a limited range of cell types. However, that does not imply that multipotent stem cells are less effective for regenerative therapies. In fact, the opposite may be true. Multipotent stem cells can differentiate into several cell types relevant to tissue repair and regeneration. For this reason, multipotent stem cells are the category of stem cells most often used in regenerative medicine.
In addition, multipotent stem cells are generally safer for therapeutic use because they are less likely to form teratomas. Furthermore, unlike totipotent and pluripotent stem cells, multipotent stem cells involve far fewer ethical concerns.
In addition to totipotent, pluripotent and multipotent stem cells, there are two other lower levels of potency. However, their use in stem cell therapy is limited.
OLIGOPOTENT
Oligopotent stem cells have a more restricted differentiation potential compared to the stem cells described above. Oligopotent stem cells differentiate into only a few closely related cell types.
Myeloid and lymphoid stem cells are classic examples of this stem cell type. These stem cells can only differentiate into cells of the blood and immune systems. Myeloid stem cells have the potential to become a type of white blood (immune) cell called granulocytes, another type of white blood cell called monocytes, and red blood cells (erythrocytes). Lymphoid stem cells have the potential to develop into lymphocytes (B and T cells) and natural killer cells, all of which are types of immune cells. The myeloid and lymphoid stem cells (as well as other oligopotent stem cells) can’t become other cell types outside of their specific group.
UNIPOTENT
As the prefix suggests, stem cells with unipotency can only differentiate into a single mature cell type. An example is germ line stem cells which can only differentiate into eggs (oocytes) and sperm (spermatozoa).
Major Types of Stem Cells
In this section, we’ll review the following primary stem cell types that are used in stem cell research and stem cell therapy:
- Embryonic stem cells (pluripotent)
- Induced pluripotent (pluripotent)
- Hematopoietic (multipotent)
- Mesenchymal (multipotent)
You may notice that totipotent stem cells are missing from this list. Totipotent cells exist only during the earliest stages of embryonic development, shortly after the sperm fertilizes the egg. Totipotency, the ability to differentiate into all cell types, may sound promising for regenerative medicine. However, totipotent cells are limited due to ethical concerns over using embryos in research. Furthermore, researchers writing in a 2022 review published in Stem Cells and Development suggest, “It is difficult to maintain the developmental totipotency and self-renewal capacity of cells cultured from early-stage embryos, which becomes a key factor limiting the research of totipotent stem cells.” [5]
Embryonic Stem Cells (ESCs)
As an embryo reaches the five-to-seven-day period after fertilization, it becomes a blastocyst consisting of a hollow sphere of cells with an inner cell mass. ESCs are derived from this inner cell mass and are pluripotent, capable of developing into any cell type in an adult body. However, ethical concerns surrounding their extraction limit their widespread use. Human ESC research uses embryos created through in vitro fertilization (IVF) procedures for reproductive purposes; the excess embryos are subsequently donated for research when no longer needed for the IVF procedure.
While embryos are still used in limited research, the next type of stem cells, also pluripotent, can be generated from adult cells, bypassing the need for embryos.
Induced Pluripotent Stem Cells (iPSCs)
iPSCs are a relatively new type of pluripotent stem cells, first generated in 2006 in a mouse by Japanese researchers at Kyoto University. iPSCs were established by introducing a small number of genes (a set of reprogramming factors) into ordinary human somatic (differentiated) cells and reprogramming them into an embryonic-like pluripotent state. [6]
Whereas human embryonic stem cells are established by removing cells from a week-old embryo and growing those cells in a culture, iPSCs can be generated using cells that are harmless to remove from an adult body. In 2015, Japanese researchers Mari Ohnuki and Kazutoshi Takahashi wrote, “…the discovery of iPSC technology has expanded the potential of regenerative medicine.” [7]
Although iPSCs avoid the ethical dilemma of destroying an embryo, their use in regenerative medicine is still in its relative infancy. Furthermore, there is concern surrounding the safety of iPSC due to the risk of tumor formation. A research review published in Stem Cells Translational Medicine explains that the “reprogramming and acquisition of stemness properties in pluripotent stem cells can also lead to the generation of malignant tumor cells.” [8]
Hematopoietic Stem Cells (HSCs)
The first successful stem cell therapy—a bone marrow transplant (BMT)—was performed by Dr. E. Donnall Thomas in 1956, using donor (allogeneic) hematopoietic stem cells. Found in the greatest quantity in bone marrow, HSCs are progenitors of blood cells, including erythrocytes—red blood cells, the primary type of blood cell responsible for oxygen transport.
Although HSCs are multipotent, meaning they can differentiate into many different types of adult cells, “they are committed exclusively to their tissue of origin, namely the hematopoietic system,” explains Albrecht M. Müller and colleagues at the Center for Experimental Molecular Medicine at the University of Würzburg, Germany. [9]
The hematopoietic system consists of the entire system dedicated to blood production (platelets, red and white blood cells, and other factors) and includes bone marrow, lymphatic organs and organelles (thymus, spleen, lymph nodes), and HSCs. Bone marrow transplants (also called hematopoietic stem cell transplantation) are primarily used to treat various blood-related disorders and cancers, such as leukemias, lymphomas, and multiple myeloma.
Research has demonstrated that the umbilical cord blood is rich in HSCs. As such, an industry has sprung up around collecting and banking the umbilical cord and umbilical cord blood for future use. Parents can choose to have their newborn’s umbilical cord blood collected within minutes after birth and cryopreserved (frozen and stored to preserve cell viability). In case a hematopoietic disease arises in the future, the HSC-rich cord blood can be used in the person whose blood was banked (autologous/same individual) to treat the disease. In some instances, the HSC derived from cord blood can be donated to a relative who’s a close genetic match to the donor (allogeneic/different individual).
HSCs are generally not used in regenerative medicine outside of blood-related disorders due to their limited potency. However, Mesenchymal Stem Cells (MSCs), another type of multipotent stem cell, have been the de facto stem cell type used in regenerative therapy over roughly the last two decades. [10]
Mesenchymal Stem Cells
Mesenchymal Stem Cells, or MSCs, hold extraordinary therapeutic potential due to their multipotent nature and inflammation-regulating properties, making them a prevalent type of stem cell used in non-blood-disorder-related stem cell therapies. In the late 1990s and early 2000s, it was thought that the ability of MSCs to differentiate into many types of somatic (mature) cells was the reason they became the cornerstone of regenerative medicine. In other words, if a knee joint needed repair, a direct injection of autologous MSCs would lead to those MSCs differentiating into healthy knee cartilage.
However, beginning in the early 2010s, researchers discovered that while the differentiation potential of MSCs contributes to their regenerative potential, it is not the only factor, nor may it be the most important factor for the regenerative effects. MSCs secrete a multitude of bioactive factors, many of which significantly modulate inflammation and other cell processes. [11]
Bioactive Factors of MSCs
Bioactive factors are a collection of signaling proteins, growth factors, and other molecules released by cells to support cell/tissue growth, development, and repair. They act like messengers, telling cells how to maintain tissue health and function.
Through bioactive factors MSCs can assist the rest of the body in a number of different ways:
- Regulate Immune Function: Depending on the inflammatory environment, MSCs can promote or inhibit an inflammatory response. For instance, MSCs can secrete either pro-inflammatory or anti-inflammatory cytokines depending on what’s needed. [12]
- Cytokines are small proteins that help control how certain immune cells act and function.
- Secretion of Anti-inflammatory Molecules: MSCs release molecules, like cytokines and growth factors, that regulate inflammation. These factors can have anti-inflammatory properties and can inhibit the activation and function of immune cells, such as T cells, B cells, and macrophages, thereby taming a hyperinflammatory response. [12]
- Angiogenesis: MSCs may secrete factors, like vascular endothelial growth factor (VEGF), that help form new blood vessels (angiogenesis). Fresh blood flow improves the delivery of oxygen and nutrients to damaged tissues. [13]
- Anti-Fibrotic Secretion: MSCs can reduce or prevent fibrosis (the development on nonfunctional scar tissue) by releasing antifibrotic factors. Unregulated fibrosis can hinder a tissue’s ability to function normally. [14]
- Reduce Oxidative Stress: MSCs can secrete endogenous (inside the body) antioxidants such as glutathione (the body’s master antioxidant). This can help reverse or manage tissue-damaging oxidative stress. [15]
The release of these bioactive molecules significantly contributes to the therapeutic potential of MSCs in helping to reduce inflammation and normalize immune responses for a wide range of medical conditions. (For this reason, Dr. Arnold Caplan, widely regarded as the “father of MSCs,” proposed in 2017 that the acronym MSCs be revised to stand for “Medicinal Signaling Cells.”) [16]
However, bioactive factors aren’t the only property that makes MSCs invaluable in regenerative medicine.
Homing
The benefits resulting from MSCs’ bioactive factors may not be as significant if they ended up in the wrong part of the body. For example, if somebody with an osteoarthritic knee received stem cell therapy but the MSCs only modulated inflammatory responses in, say, the elbow, the procedure to repair damaged knee cartilage may have limited effects. [17]
However, MSCs know exactly where they are needed most in the body, thanks to a process called homing. Homing is the process by which stem cells find and travel to a specific destination. This ability enables MSCs to locate areas of the body where pro-inflammatory signaling occurs. [18, 19]
Homing allows for stem cell therapy to be administered intravenously. For example, IV MSC therapy has been found to help improve neurological deficits following stroke. [20] Following IV administration, a number of the infused MSCs travel through the bloodstream to the damaged, inflamed tissue site, thanks to homing.
The Regenerative Potential of MSCs Cultured by Celltex Therapeutics
MSC therapy has emerged as a potential intervention for several conditions, with the most promising results thus far demonstrated in osteoarthritis, soft-tissue and joint injuries, and autoimmune conditions. However, clinical trials have shown its potential in addressing a variety of other conditions as well, including mood disorders, cardiovascular repair, gastroenterological diseases, neurological conditions, and pulmonary disorders.
Although you already have stem cells in your body, the number of stem cells in most body tissues will decline as you age. Furthermore, stem cells will lose potency over time, although several factors may influence the degree of this decline.
Eventually, the number of stem cells in one’s body may not be enough to address chronic conditions, serious injuries, or complications that often come with aging. That’s why banking your stem cells with Celltex is recommended.
Considering Stem Cell Therapy? Bank Your MSCs with Celltex First
Celltex Therapeutics is revolutionizing regenerative medicine with the process of Adult Stem Cell Banking.
Adult Stem Cell Banking is the critical first step in the stem cell therapy process that separates Celltex from other regenerative medicine options.
Adult Stem Cell Banking with Celltex involves:
1. A simple, one-time extraction (mini liposuction) of an individual’s fat tissue
2. Isolating the MSCs from that tissue sample
3. Expanding the pure MSCs in quantity
4. Cryopreserving a lifetime supply for future therapeutic uses
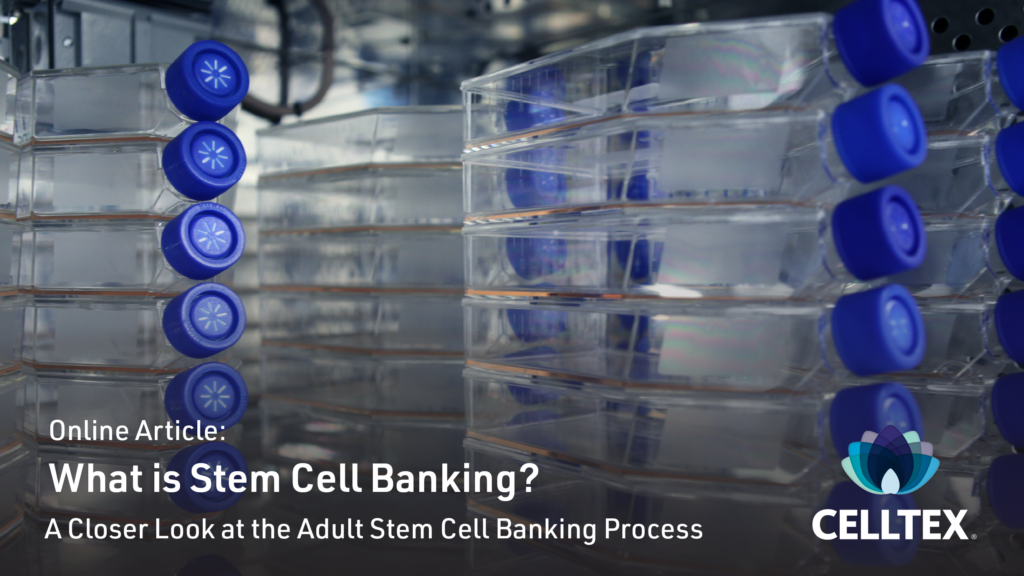
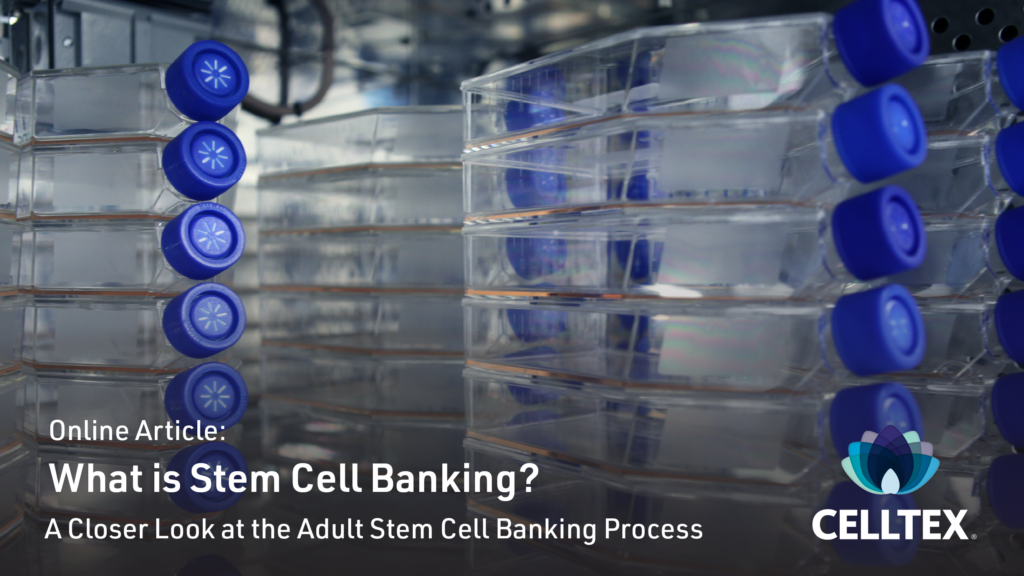
Celltex’s technology enables an individual’s banked MSCs to be expanded into quantities exponentially greater than that of other regenerative medicine offerings, greatly increasing the potential for positive results when therapeutic application is necessary.
This difference in cell quantity, along with the compatibility from using one’s own cells contributes to the superior quality of Celltex-produced MSCs. Celltex-produced MSCs have the potential to succeed where other stem cell therapies may have failed before.
Learn More About Celltex
Founded by entrepreneur David G. Eller and world-renowned spine surgeon Dr. Stanley C. Jones, Celltex is able to isolate, expand, and cryopreserve your own adipose-derived MSCs. Celltex-cultured MSCs have been used in over 10,000 stem cell therapies with no severe adverse events.
Interested in learning more about Celltex Adult Stem Cell Banking? Request a virtual brochure, watch our educational webinars, or contact us directly.
If you’re in the Houston area, you can schedule a tour of our lab in person or tour it virtually.
References:
- Aly R. M. (2020). Current state of stem cell-based therapies: an overview. Stem cell investigation, 7, 8. https://doi.org/10.21037/sci-2020-001
- Ghazimoradi M. H., Khalafizadeh A., & Babashah S. (2022) A critical review on induced totipotent stem cells: Types and methods. Stem Cell Research 63. https://doi.org/10.1016/j.scr.2022.102857
- Du P., & Wu J. (2024) Hallmarks of totipotent and pluripotent stem cell states. Cell Stem Cell, 31(3), 312-333. https://doi.org/10.1016/j.stem.2024.01.009
- Damjanov, I., & Andrews, P. W. (2016). Teratomas produced from human pluripotent stem cells xenografted into immunodeficient mice – a histopathology atlas. The International journal of developmental biology, 60(10-11-12), 337–419. https://doi.org/10.1387/ijdb.160274id
- Cai, J., Chen, H., Xie, S., Hu, Z., & Bai, Y. (2022). Research Progress of Totipotent Stem Cells. Stem cells and development, 31(13-14), 335–345. https://doi.org/10.1089/scd.2022.0061
- Aboul-Soud, M. A. M., Alzahrani, A. J., & Mahmoud, A. (2021). Induced Pluripotent Stem Cells (iPSCs)-Roles in Regenerative Therapies, Disease Modelling and Drug Screening. Cells, 10(9), 2319. https://doi.org/10.3390/cells10092319
- Ohnuki, M., & Takahashi, K. (2015). Present and future challenges of induced pluripotent stem cells. Philosophical transactions of the Royal Society of London. Series B, Biological sciences, 370(1680), 20140367. https://doi.org/10.1098/rstb.2014.0367
- Iglesias, J. M., Gumuzio, J., & Martin, A. G. (2017). Linking Pluripotency Reprogramming and Cancer. Stem cells translational medicine, 6(2), 335–339. https://doi.org/10.5966/sctm.2015-0225
- Müller, A. M., Huppertz, S., & Henschler, R. (2016). Hematopoietic Stem Cells in Regenerative Medicine: Astray or on the Path?. Transfusion medicine and hemotherapy : offizielles Organ der Deutschen Gesellschaft fur Transfusionsmedizin und Immunhamatologie, 43(4), 247–254. https://doi.org/10.1159/000447748
- Ramaswamy Reddy, S. H., Reddy, R., Babu, N. C., & Ashok, G. N. (2018). Stem-cell therapy and platelet-rich plasma in regenerative medicines: A review on pros and cons of the technologies. Journal of oral and maxillofacial pathology : JOMFP, 22(3), 367–374. https://doi.org/10.4103/jomfp.JOMFP_93_18
- Hofer, H. R., & Tuan, R. S. (2016). Secreted trophic factors of mesenchymal stem cells support neurovascular and musculoskeletal therapies. Stem cell research & therapy, 7(1), 131. https://doi.org/10.1186/s13287-016-0394-0
- Zhou, C., & Bai, X. Y. (2022). Strategies for the induction of anti-inflammatory mesenchymal stem cells and their application in the treatment of immune-related nephropathy. Frontiers in medicine, 9, 891065. https://doi.org/10.3389/fmed.2022.891065
- Kasper, G., Dankert, N., Tuischer, J., Hoeft, M., Gaber, T., Glaeser, J. D., Zander, D., Tschirschmann, M., Thompson, M., Matziolis, G., & Duda, G. N. (2007). Mesenchymal stem cells regulate angiogenesis according to their mechanical environment. Stem cells (Dayton, Ohio), 25(4), 903–910. https://doi.org/10.1634/stemcells.2006-0432
- Dong, L. H., Jiang, Y. Y., Liu, Y. J., Cui, S., Xia, C. C., Qu, C., Jiang, X., Qu, Y. Q., Chang, P. Y., & Liu, F. (2015). The anti-fibrotic effects of mesenchymal stem cells on irradiated lungs via stimulating endogenous secretion of HGF and PGE2. Scientific reports, 5, 8713. https://doi.org/10.1038/srep08713
- Stavely, R., & Nurgali, K. (2020). The emerging antioxidant paradigm of mesenchymal stem cell therapy. Stem cells translational medicine, 9(9), 985–1006. https://doi.org/10.1002/sctm.19-0446
- Caplan A. I. (2017). Mesenchymal Stem Cells: Time to Change the Name!. Stem cells translational medicine, 6(6), 1445–1451. https://doi.org/10.1002/sctm.17-0051
- Freitag, J., Bates, D., Wickham, J., Shah, K., Huguenin, L., Tenen, A., Paterson, K., & Boyd, R. (2019). Adipose-derived mesenchymal stem cell therapy in the treatment of knee osteoarthritis: a randomized controlled trial. Regenerative medicine, 14(3), 213–230. https://doi.org/10.2217/rme-2018-0161
- Fan, D., Xie, X., Qi, P., Yang, X., & Jin, X. (2017). Human mesenchymal stem cell homing induced by SKOV3 cells. American journal of translational research, 9(2), 230–246.
- Ullah, M., Liu, D. D., & Thakor, A. S. (2019). Mesenchymal Stromal Cell Homing: Mechanisms and Strategies for Improvement. iScience, 15, 421–438. https://doi.org/10.1016/j.isci.2019.05.004
- Andrzejewska, A., Dabrowska, S., Lukomska, B., & Janowski, M. (2021). Mesenchymal Stem Cells for Neurological Disorders. Advanced science (Weinheim, Baden-Wurttemberg, Germany), 8(7), 2002944. https://doi.org/10.1002/advs.202002944
Post Tags: medical research, Research, stem cell banking, stem cell therapy
More Recent News
Stem Cell Therapy: How Are MSCs Administered to the Body?
Through the years, several stem cell therapy delivery methods have emerged. This article explores some of the most common routes of administration physicians may recommend today.
Read MoreThe Bryan-College Station Eagle: Celltex’s Jackie Sherrill Featured as a Guest Columnist to Comment on the Upcoming Texas A&M vs. Texas Game
Coach Jackie Sherrill, President of Celltex Sports Biologics, was featured as a guest columnist in The Bryan-College Station Eagle to share his excitement for the renewed rivalry game between Texas A&M University and the University of Texas.
During Sherrill’s tenure as Texas A&M’s head football coach from 1982 to 1988, the team went 5-2 against Texas. In the article, Coach Sherrill reflects on the past rivalry games and shares what he believes to be the key to winning this year’s Lone Star Showdown.
Read More